Using Isolators and Bio-Decontamination to Future-Proof CGT Processes and Facilities

The production of Advanced Therapy Medicinal Products (ATMPs) can have many complex manual steps, which necessitates meticulous aseptic processing conditions to ensure the product is sterile, which is critical for patient safety. Closed isolator systems provide a consistent, compliant, and cost-effective solution, and can play a critical role in ensuring the safety of ATMPs.
To further the understanding of biopharmaceutical or human cell therapy process development to include improvements of advanced, automated technologies and digital monitoring (e.g., Pharma 4.0™), the following objectives should serve as constant criteria: patient safety, access to therapies, scalability, speed of production, and compliance with current GMPs (GMPs). To meet these criteria, a steady and repeatable strategy is essential.
To reduce the risk of contamination, the industry needs to work toward the following:
- Closed process design
- Thorough understanding of where human operators interact with the process
- Reduction of human operator interaction in critical areas of the process
- A contamination control strategy that encompasses process, equipment, people, and facilities with risk-mitigating approaches
Expertise and risk assessment can set a foundation for evaluating and making ongoing improvements to ensure patient, product, and business risks are mitigated. The following discussion will offer options for approaches to contamination control using technology, expertise, and a culture of quality improvement. These options have historical experience in successfully handling biopharmaceutical and cell therapy materials.
Rethinking Phase Appropriate Compliance
Current Guidance
For this industry, current GMPs are constantly under scrutiny to stay up-to-date with new technology. Some guidance and GMPs have global impact. Specifically:
- Pharmaceutical Inspection Convention/Pharmaceutical Inspection Co-operation Scheme (PIC/S): Annex 2A, Manufacture of Advanced Therapy Medicinal Products for Human Use to Guide for Good Manufacturing Practice for Medicinal Products1
- The European Commission:
- EudraLex, Volume 4: Good Manufacturing Practice (GMP) guidelines. Part IV: Guidelines on Good Manufacturing Practice specific to Advanced Therapy Medicinal Products 2
- EudraLex Volume 4: EU Guidelines for Good Manufacturing Practice for Medicinal Products for Human and Veterinary Use. Annex 1: Manufacture of Sterile Medicinal Products 3
- EudraLex Volume 4: EU Guidelines for Good Manufacturing Practice for Medicinal Products for Human and Veterinary Use. Annex 2: Manufacture of Biological Active Substances and Medicinal Products for Human Use 4
- The US Food and Drug Administration:
- International Council for Harmonization of Technical Requirements for Pharmaceuticals for Human Use, ICH Harmonized Guideline. Q9 (R1): Quality Risk Management 7 (this has become the reference approach for quality risk management justification)
- Other regional guidance has its own versions of the latter regulatory perspectives.
Sterility Assurance and Contamination Control
Aseptic manufacturing has always been a highly scrutinized process. In some cases, the lack of a product sterilization step (e.g., in cell therapy because cells are too large to pass through a sterile filter) has led to high-risk concern for assuring proper, robust processes and procedural design for contamination control. The common sources of contamination in aseptic manufacturing are people, materials and equipment, and the environment. The risk assessment approach can map the potential origins of microbial contamination, determine the level of risk to mitigate, and holistically examine the integration of process steps, along with the design of equipment and cleanroom space, to decide how to mitigate the risks.
From a microbiological perspective, contamination control spans from small-scale manufacturing for clinical trials to larger-scale approved commercial manufacturing. Compliance with GMPs and a contamination control strategy are essential at all phases. Appropriate microbiological validation (qualification) is a fundamental requirement, in contrast to other types of validation development, to ensure no harm to the patient. The earlier an automated system can be included in process development, the less validation and change management will be required during scale up to commercial approval.
Design of a process and the cleanroom space surrounding it becomes a significant factor to sterility assurance. Closed systems, robust material transfer, and separation of operators from product within classified areas are design parameters to consider. Complexity of design can lead to higher costs. Considerations for the latter will influence the cost of goods in the long run (i.e., from clinical manufacturing to commercial-scale manufacturing). Leading industry organizations, such as ISPE, have encouraged manufacturers to view isolators as an appropriate new containment design consideration for aseptic processes (including sterility testing) that could replace the use of open biological safety cabinets and laminar air hoods, where possible.
A balance between manual processing and automated processing is possible with currently available technology. The intended design improvements can eventually lead industry to fully automated processing. Although autologous processes are currently a combination of manual and automated (using closed instrument systems with one or more steps), scientists are attempting to develop allogeneic processes in platform approaches for consistency of the larger-scale volumes. Depending on the location of manufacturing (e.g., offsite or bedside for autologous) and volume of therapy batch (as for allogeneic), designing a fully closed system that can maintain aseptic control is a primary consideration. Progression from clinical to commercial should consider ways to minimize changes in design and maintain consistency in contamination control.
It is important and valuable to consider options for a critical process, such as cleaning and bio-decontamination, because your choice will influence both contamination control and the cost of goods. Cleaning and bio-decontamination is more than just a routine program. These processes consist of selecting effective, compatible, and regulatory compliant cleaning and disinfecting agents, proper and relevant application of the chemical agents, and application at an appropriate frequency to meet efficacy and contamination control requirements. Manual cleaning and disinfection have inherent variability, which should be supplemented by more consistent, validated approaches, where possible. This is where options for improvement by automation using hydrogen peroxide vapor should be a strong consideration, for equipment, process, and cleanroom spaces.
ISOLATOR ADVANTAGES FOR CLOSED SYSTEMS
The production of ATMPs can have many complex manual steps, which necessitates meticulous aseptic processing conditions to ensure patient safety and product efficacy. Although traditional open systems like laminar flow hoods (LFHs) and biosafety cabinets (BSCs) have found widespread application due to their low cost and ease of use, these systems possess inherent limitations. The advantages of closed isolator systems as a compelling alternative for ATMP manufacturing are emphasized by their ability to enhance contamination control and streamline production processes.
OPEN SYSTEMS LIMITATIONS
Open systems, although effective to a certain extent, rely on rigorous operator aseptic techniques and stringent cleanroom maintenance for contamination control. This translates to increased operational costs due to multiple layers of operator gowning, energy consumption from the heating, ventilation, and air conditioning systems, additional environmental monitoring, and frequent cleanroom decontamination cycles. In addition, the inherent openness of these systems necessitates constant vigilance to prevent airborne contaminants from compromising product sterility.
CLOSED ISOLATOR SYSTEMS: A PARADIGM SHIFT
Isolator systems offer a transformative approach to ATMP manufacturing. They function as self-contained units, physically separating the critical processing zone from the operator environment. This physical barrier significantly reduces the risk of contamination from human intervention. In fact, one particular study from an autologous cell therapy manufacturer that was using both LFHs and isolators to produce their product found that the batch failure rate in the LFH was 10%, whereas in the isolator it was only 3%9. Furthermore, isolators utilize a cascaded pressure differential system similar to cleanroom design principles, as well as unidirectional airflow over the product/process. This creates a controlled aseptic zone within the isolator, minimizing the influence of external contaminants.
Isolators can be hosted in a lower-grade cleanroom than an open LFH or BSC. This is evidenced by EU GMP Annex 1, which states in section 4.4 that grade B is required as the background cleanroom for grade A (where it is not an isolator), i.e., an LFH or BSC, and that grade C and D is the background for isolators3. Downgrading from a grade B to grade C/D cleanroom results in substantial cost savings from reduced gowning, environmental monitoring, cleaning and disinfection, and energy consumption. In fact, one study which investigated the running costs between different cleanroom grades found that grade C/D cleanrooms resulted in 30% lower running costs than grade B cleanrooms 10.
ENHANCING ASEPTIC PROCESSING WITH INTEGRATED TECHNOLOGIES
Modern isolator systems are equipped with advanced features that bolster aseptic processing. This includes being integrated with hydrogen peroxide vapor (H2O2) bio-decontamination technology. This automated process demonstrably achieves a 6-log sporicidal reduction in bioburden on the isolator chamber surfaces as well as the surfaces of incoming materials, ensuring a high degree of microbial inactivation prior to product introduction.
The bio-decontamination process consists of four distinct steps:
- Conditioning: The hydrogen peroxide vaporizer heats up to the necessary operating temperature for effective vaporization, and the instruments being decontaminated warm up and stabilize ensuring they are ready for the next steps.
- Gassing: Hydrogen peroxide liquid is vaporized and dispersed throughout the chamber. The vapor reaches the dew point and micro-condensation forms on surfaces.
- Dwell: The hydrogen peroxide vapor is either maintained at a constant level or the vapor is distributed without additional vapor.
- Aeration: The hydrogen peroxide vapor is catalytically converted into water vapor and oxygen.
The bio-decontamination process outlined previously is typically validated with Geobacillus stearothermophilus biological indicators (BIs), which are placed at strategic challenge locations within the isolator (i.e., corners, equipment, hot/cold locations, and filter faces). Furthermore, calibrated chemical indicators can be used to verify cycle performance on an ongoing basis.
In the ATMP sector, not all incoming materials can undergo hydrogen peroxide vapor bio-decontamination. Due to its small molecule size hydrogen peroxide can permeate through some bags. This is generally a consideration for any living material such as the cells being processed because they can be damaged or even killed by the hydrogen peroxide. For such sensitive living materials, alternative methods may need to be explored to transfer them into the isolator. This topic will be explored later in the article.
Additionally, isolator systems can be seamlessly integrated with other technologies, such as environmental monitoring (EM) equipment, audit trail software to support compliance with 21 CFR Part 11 8, and glove integrity test equipment to further provide peace of mind on the operations conducted within. EM typically consists of two components. The first is an integrated viable air sampler that draws a validated volume of air from the isolator over an agar plate to collect any airborne microorganisms that may be present. The second is a continuous particle monitoring system, which has three main elements: an air feed from the isolator chamber via an isokinetic cone, a particle counter through which the extracted air is passed, and a computer to log the particle counter operation and results.
When combined with standard operating procedures, the comprehensive approach of using isolators for aseptic processing provides a robust framework for maintaining aseptic conditions throughout the ATMP manufacturing process.
Streamlining Workflows Within a Closed System
While ATMP processing within an isolator will vary depending on the application, a typical workflow generally involves a multi-stage decontamination process. Initially, the entire isolator system undergoes a bio-decontamination cycle with no materials inside. This “system empty cycle” targets the chamber surfaces and achieves a validated 6-log bio-decontamination. This cycle focuses on high-frequency touchpoints like gloves, sleeves, chamber floors, and racking, minimizing potential contamination sources.
Following the system bio-decontamination, necessary tools and materials are transferred into the isolator via a designated transfer chamber. These tools and materials then undergo a dedicated rapid bio-decontamination cycle tailored to the specific material loading being introduced. This may en-compass flasks, pipettes, agar plates, and other process-specific items. These items should be hung on racking to maximize exposure to the hydrogen peroxide vapor.
Finally, after both the isolator and incoming materials have been bio-decontaminated, the living/cellular material is introduced into the chamber via an alternative material transfer method to avoid any exposure to hydrogen peroxide vapor, which could adversely impact it. Depending on the process, this may involve rapid transfer ports, liquid transfer ports, or manual transfer via a dedicated airlock and manual disinfection of the outer surfaces.
The use of these transfer mechanisms minimizes operator intervention and the associated risk of contamination. All materials will be transferred into a working chamber where the aseptic process will be conducted, and an additional airlock can be used to allow egress of materials without losing the aseptic conditions within the working chamber. The diagram in Figure 1 outlines the typical workflow described.
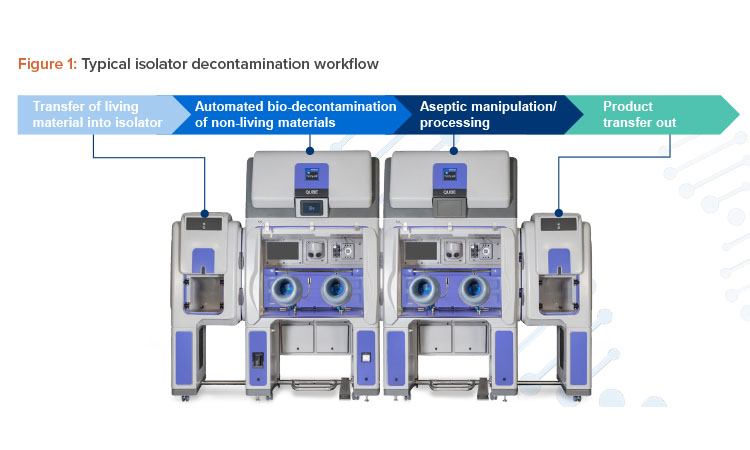
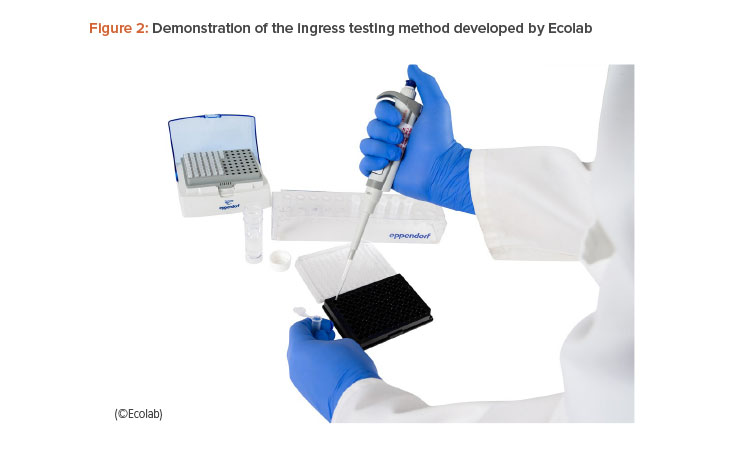
Addressing Potential Concerns
Although the advantages of isolator systems are clear, some initial hesitation regarding their implementation is understandable. Cost is a common concern. However, advancements in isolator technology have made them more budget-friendly and the initial cost can be offset by the reduced running costs that are delivered by operating a lower-grade cleanroom.
Additionally, some isolators are designed for easy integration into existing spaces without room modifications and can be configured to fit the space constraints that may have previously prevented their use. Isolator manufacturer’s validation engineers can validate isolators in days instead of weeks, speeding up system use and reducing the burden on site staff. A potential concern is working through glove ports. But as technology evolves, glove systems become more lightweight and easier to work with.
Another concern, which was already outlined, is the robust transfer of living materials (cells) into isolators using a method that will not damage the cells or bring contamination into the aseptic environment. Before exploring other transfer methods, it’s necessary to first establish whether the ideal method of bio-decontaminating with hydrogen peroxide vapor risks penetrating the container housing living materials and damaging them. This can be determined by performing ingress testing. The process involves running de-ionized water through the entire procedure to be performed in the isolator, in place of the drug product, and then measuring the levels of hydrogen peroxide (if any) in the de-ionized water.
Using a horseradish peroxidase (HRP)-based assay, levels in the water as low as 15 parts per billion (ppb) can be detected. If no hydrogen peroxide is detected at these low levels, it provides evidence that there is no ingress of hydrogen peroxide during the bio-decontamination process. Thus, the living material is highly unlikely to be detrimentally affected. Ingress testing should be performed using the same load and preferably the same isolator system in which the aseptic process is taking place to accurately simulate the process.
If testing reveals significant ingress into the container housing the living materials, alternative material transfer methods should be used to minimize contamination risk in the aseptic environment and reduce the risk of damage to the living material.
Conclusion
Closed isolator systems represent a future-proof strategy for ATMP manufacturers seeking to enhance contamination control and streamline aseptic production processes. By offering a consistent, compliant, and cost-effective solution, isolators can play a critical role in ensuring the safety and efficacy of ATMPs. This will help pave the way for advancements in this rapidly evolving field.